how direct measurements can be improved to successfully incorporate into voluntary carbon markets.
Voluntary carbon markets can often feel like the wild west, where the voluntary nature of the markets enables programs to establish their own standards. However, for soil carbon markets, the fundamental goal is shared across all programs: to quantify changes in soil carbon. While it may seem straightforward, accurately estimating changes in soil carbon stock can be a challenging task. At Nori, we approach this by estimating the changes in soil organic carbon using biogeochemical modeling that is calibrated and validated by soil samples. Of course there are other approaches to this task, and settling on the most appropriate methods for use in a carbon market is anything but simple.
There are two main schools of thought behind soil carbon estimation: direct measurement or biogeochemical modeling. At Nori, we believe that the future of carbon markets will include direct measurement estimations of carbon. However, these direct measurements need to be made standardized, scalable, and accessible enough to be meaningfully incorporated into voluntary carbon markets. For now, we depend on biogeochemical modeling to inform our carbon removal offsets, and we are working towards developing accessible solutions for integration of project-scale soil sampling.
This article explains:
- The common means of direct soil measurement and the challenges in this approach;
- Why Nori currently uses biogeochemical modeling to achieve scientifically sound estimates that enable flexibility to scale; and
- What we believe needs to be improved upon to allow direct measurement to be successfully incorporated into voluntary carbon markets.
Estimating Soil Carbon
The direct measurement of soil organic carbon is most commonly achieved through soil samples taken directly from fields to build site-specific estimates of soil organic carbon stock. Sampling soil for carbon is often compared to sampling for soil nutrients: pulling a few composite cores each fall, sending them to the lab, and making recommendations from the results. Decades of research have defined the “how and why” of nutrient sampling. Nutrient sampling has the benefit of a standardized methodology, from how deep to pull cores, to which assays are used to analyze nutrient compositions, an understanding of nutrient usage and movement in the soil profile, and accessibility for all types of farmers and agronomists to pull these samples anywhere throughout croplands. The result is simple: if numbers fall within a given range, apply “x” amount of fertilizer.
In contrast, soil sampling for carbon sequestration does not have a widely-accepted standard methodology*, yet. A wide range of sampling approaches have been developed by several organizations involved in carbon markets such as Verra, Climate Action Reserve, Australian Clean Energy Regulator, etc. Within this ecosystem of approaches, there are some common threads of standardized techniques that have emerged from research, such as defined tests for measurement (e.g. dry combustion, loss on ignition, etc.)¹, and important details like measuring bulk density to understand scale². Soil sampling using these techniques in research and in practice has progressed understanding of soil carbon dynamics and informed modeling efforts. However, some of the research techniques, such as setting aside control fields, are not easily transferable to practice within carbon markets. For accessible markets, understanding of the uncertainties and the effects of agronomic practices on soil carbon dynamics need to be leveraged to minimize the financial costs, time, and land usage.
Quantifying organic carbon stock change in a given field can be resource-intensive due to the high sample-to-sample variability across the field. This natural background variability is almost always greater than the increase in organic carbon that is being measured, resulting in a challenging task of “separating the signal from the noise”. To illustrate, Figure 1 shows idealized distributions of organic carbon at a field before and after 10 years of regenerative practices. In this simplified example, we are assuming realistic values of 0.3 weight percent standard deviation within the field³ ⁴ ⁵, with a 0.6 tonnes of CO2 sequestration per acre per year. As you may suspect based on this idealized case, it would require many soil samples to detect a change in organic carbon stock, even after 10 years.
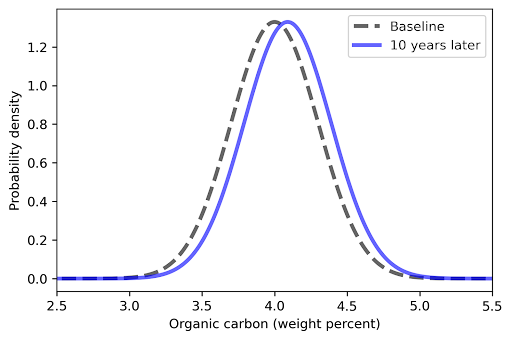
To put some solid numbers behind that, we can calculate the number of samples needed to robustly estimate the change in organic carbon stock of a field, given the above assumptions. This number can be large, and increases if greater certainty is required. Figure 2 illustrates the number of samples needed to constrain the uncertainty in detecting the “signal”. One piece of information this analysis gives us is that in order to have a 90% chance of detecting ANY increase in organic carbon content after 10 years of regenerative practices, at least ~40 samples are needed for the baseline and another ~40 samples for the final sampling. Said another way, even with 40 samples per sampling event, direct measurement could falsely indicate a decrease in organic carbon stock about 10% of the time. And the number of samples needed to more precisely determine the actual increase in carbon content would be even greater. While there are significant benefits to storing carbon in soil, this analysis demonstrates the difficulty in accurately measuring these changes in natural environments.
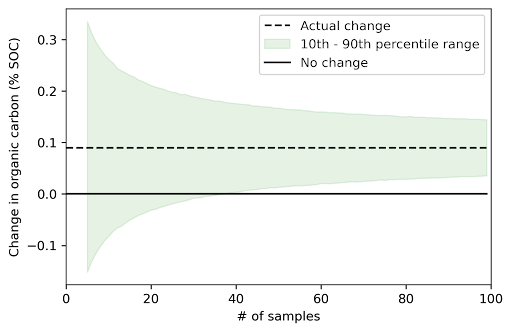
Notice that the size of the field was not specified or needed for this analysis. The number of samples needed is not directly related to the size of the field, only to the variability within the field. However with increasing field size, the in-field variability may also increase due to a range of factors. Besides natural background variability, factors such as soil type, management practices, slope, and elevation can have a major effect on the organic carbon content of any given field. However, this knowledge can actually help guide sampling by separating the sampling area into multiple sections based on these factors, which is commonly referred to as “stratified sampling”. This type of sampling can be applied to a single field or to multiple nearby fields that share the same characteristics.
Stratified sampling, combined with other techniques such as composite sampling, in-situ sampling, and remote sensing can potentially be incorporated into a sampling strategy to reduce the costs of sampling while improving the accuracy of the organic carbon stock estimates. Nori is currently exploring the available technologies to evaluate their applicability to soil carbon markets.
A scalable soil sampling protocol will need to be designed with intention to address the needs of the soil carbon market, Nori won’t simply add soil sampling to check a box. We recognize the importance of soil sampling in establishing a strong and sustainable voluntary marketplace, but before incorporating such an option into our approach, we must ensure a reliable and accessible design that supports the scaling up of regenerative agriculture practices.
Quantification approach
Given that we don’t yet incorporate direct measurements of carbon into our US Croplands Methodology, how does Nori currently use biogeochemical modeling to create reputable, science-backed carbon removal offsets? In sum:
- We are very conservative in our quantification; and
- Our carbon removal offsets have a reasonable length of time that the carbon will remain in the ground.
The science is clear⁶ that soil carbon does not accumulate linearly and is highly dependent on annual weather. Therefore, multiple years of data are needed to robustly estimate trends and avoid over-issuing carbon removal offsets based on just a few years of carbon gains. At this stage, data-backed biogeochemical modeling is our best tool for understanding these carbon trends and building scalable carbon markets. We use a process-based modeling platform that meets IPCC Tier 3 and USDA Blue Book standards for soil organic carbon accounting, and utilizes high-resolution soil and weather data from SSURGO and NOAA, respectively.
Modeling allows us to estimate the effects of changing agronomics on soil carbon dynamics over time. We acknowledge that modeling tools cannot fully capture the intricacies of modern farming, but they have been a good starting point. Modeling as our initial carbon quantification tool has kept Nori in line with carbon accounting standards , while promoting a farmer forward outlook by scalably quantifying carbon removal and making carbon markets accessible to farmers for their regenerative, stewardship-minded practices.
There is a level of uncertainty with any modeling tool, so Nori takes a conservative approach to quantification. To account for model uncertainties, our issuances are based on soil organic stock gains that are about 30% less, on average, than what the model predicts as the annual average on a per project basis. Our projects average about 0.6 tonnes of CO2 sequestered per acre per year, which is consistent with the academic understanding of carbon flux gains in the topsoil of agricultural land⁷ ⁸.
Nori will stand behind the transaction between buyer and supplier to ensure our product is held to clear, achievable, and high standards. Launching our cryptocurrency token — the $NORI — will create a built-in insurance pool of reserved tokens to use— to make the buyer whole— in the event our projects have premature carbon reversal or we find that we over-estimated carbon sequestration using biogeochemical modeling. Our carbon removal offset, the Nori Regenerative Tonne, represents one tonne of CO2 removed from the atmosphere and retained in a reservoir (soil, in this case) for a minimum of 10 years. Each supplier signs our Regenerative Tonne Agreement allowing us to ensure that the carbon sold stays in the ground for a minimum of 10 years. We define a 10 year period of carbon retention to strike a balance between carbon retention in the soil and the practicality of farmers’ management timescales on their land.
Both our insurance pool and Regenerative Tonne agreement ensure that the purchaser of the carbon removal offset has full guarantee that the carbon they purchase is held to our clear standards: 1 Nori Regenerative Tonne is equivalent to 1 tonne CO2 removed from the atmosphere for a minimum of 10 years.
How Soil Sampling Can Scale
Nori has designed its system to be accessible to as many farmers as possible, and we aim to minimize the financial burden of any long-term soil sampling. With this in mind, we also see incorporation of soil sampling as an opportunity to enhance the certainty and increase the understanding of changes in soil organic carbon stocks. We believe that recent regenerative agriculture research can be leveraged to develop a scientifically-robust and financially accessible framework for incorporating soil sampling into soil carbon stock tracking.
On the research side, the amount of grant funding towards soil carbon studies and regenerative agriculture is increasing, with hundreds of millions of dollars from the USDA alone being invested in soil health and climate smart agriculture research⁹ ¹⁰. From this recent soil carbon research, we have already learned how to better stratify soil sampling in ways that are more accessible to a full farm audit¹¹ and the development of frameworks to aggregate and standardize soil sampling for those outside academia¹².
Incorporating new developments and technologies into the carbon market space will need three things:
- Standardization — A carbon removal offset at Nori should equal a carbon removal offset anywhere and we need a clear understanding of the data quality so that our tools minimize uncertainty, rather than add to it.
- Scalability — Gathering ground-truthed data can’t reasonably operate like a replicated experimental design. Taking the understanding we have from the scaling of the nutrient sampling industry can set the stage for scaling carbon sampling. If we learn from that, we can scale more effectively, sustainably-minded, and farmer-focused.
- Accessibility — The cost of long-term soil carbon sampling needs to be economically feasible within the carbon market.
Nori is eager to incorporate soil sampling into our carbon marketplace with science and tools that are standardized, scalable, and accessible. For now, we estimate carbon in the most scalable standard method available—biogeochemical modeling. As we continue to progress and evolve with advancements in science, we are actively evaluating innovative new ways to integrate direct measurement of soil carbon into the marketplace.
Note: This is an update to the blog post originally published on February 9th, 2022.
References
- A comparison of some methods for soil organic carbon determination by Y.K. Soon and S. Abboud
- https://soilhealthlab.cals.cornell.edu/testing-services/soil-health-analysis-packages/
- Soil Resources, Microbial Activity, and Primary Production Across an Agricultural Ecosystem by Robertson, G. P., K. M. Klingensmith, M. J. Klug, E. A. Paul, J. R. Crum, and B. G. Ellis
- Optimization for Estimating Soil Organic Carbon Stocks in Agroecosystems by Sherpa, S. R., D. W. Wolfe, and H. M. van Es
- How to estimate soil organic carbon stocks of agricultural fields? Perspectives using ex-ante evaluation by Potash, E.; Guan, K.; Margenot, A.; Lee, D.; DeLucia, E.; Wang, S.; Jang, C
- A review of soil carbon dynamics resulting from agricultural practices by Farhat Abbas, Hafiz Mohkum Hammad, Wajid Ishaq, Aitazaz Ahsan Farooque, Hafiz Faiq Bakhat, Zahida Zia, Shah Fahad, Wajid Farhad, and Artemi Cerdà
- Management of cover crops in temperate climates influences soil organic carbon stocks: a meta-analysis by Shelby C. McClelland, Keith Paustian, and Meagan E. Schipanski
- How does tillage intensity affect soil organic carbon? A systematic review by Neal R. Haddaway, Katarina Hedlund, Louise E. Jackson, Thomas Kätterer, Emanuele Lugato, Ingrid K. Thomsen, Helene B. Jørgensen, and Per‑Erik Isberg
- USDA Invests $21.7M in Research Innovations to Improve Soil Health and Climate Smart Agriculture and Forestry (United States Department of Agriculture)
- Partnerships for Climate-Smart Commodities (United States Department of Agriculture)
- Farm-scale soil carbon auditing by J.J. de Gruijter, A.B. McBratney, B. Minasny, I. Wheeler, B.P. Malone, U. Stockmann
- Soil organic carbon is not just for soil scientists: measurement recommendations for diverse practitioners by S. A. Billings, K. Lajtha, A. Malhotra, A. A. Berhe,M.-A. de Graaff, S. Earl,J. Fraterrigo, K. Georgiou,S. Grandy, S. E. Hobbie, J. A. M. Moore, K. Nadelhoffer, D. Pierson,C. Rasmussen, W. L. Silver, B. N. Sulman, S. Weintraub, W. Wieder